Rizo lab
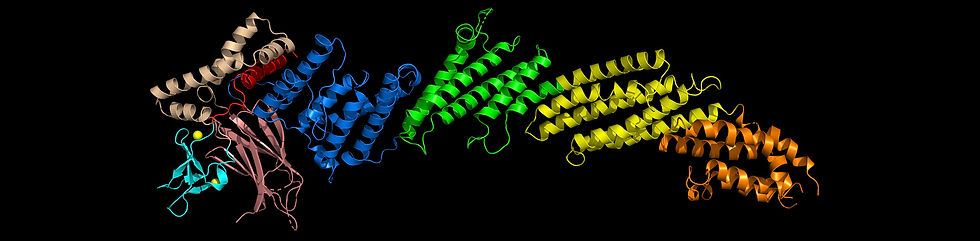
Neurotransmitter release by synaptic vesicle exocytosis constitutes an exquisitely regulated form of membrane fusion. Each molecular event that changes the release probability provides a means to process information in the brain. Hence, a key challenge in this field is not only to determine the basic mechanism of membrane fusion but, perhaps even more important, to elucidate how fusion is regulated during a wide variety of presynaptic plasticity processes that enable in part the many wonders of the human brain.
Release is restricted to specialized areas of the plasma membrane called active zones, which are formed by very large, multidomain proteins and enable many forms of regulation of release. Particularly important among these proteins are: i) Munc13s, which mediate multiple regulatory processes through their various domains, in addition to being essential for release; and ii) RIMs, which provide a scaffold to organize the active zone and mediate tethering of synaptic vesicles to the active zone by binding to Rab3s, which are small GTPases localized on synaptic vesicles (reviewed in ref. 1). Panel A on the right shows the domain structure of Munc13-1 and RIM1. The functions of Munc13-1 and RIMs are intimately connected through binding of the RIM ZF domain to the Munc13-1 C2A domain [2, 3].
We have dedicated major efforts to characterize the structure and biochemical properties of Munc13-1 and RIMs to provide a framework to understand their regulatory roles and to correlate these properties with physiological data through several collaborations. Panel B shows the structures of diverse domains of Munc13-1 and RIMs that we have determined using NMR spectroscopy or X-ray crystallography. Some of our more important contributions in this area include: i) the demonstration that Munc13-1, RIMs and Rab3s form a tripartite complex that likely couples vesicle tethering to Munc13- and RIM-dependent forms of presynaptic plasticity [3]; ii) the discovery that the Munc13-1 C2A domain forms a homodimer, which competes with its heterodimerization with the RIM ZF domain, as well as the elucidation of the structures of the homodimer and the heterodimer [4]; iii) the determination of the structure of the Munc13-1 C2B domain and the demonstration of its role in short-term presynaptic plasticity [5]; iv) the demonstration that the RIM PDZ domain binds to Ca2+ channels, thus localizing the channels near the site of fusion [6]; v) the elucidation of the structures of the RIM C2 domains [7, 8] and the finding that these domains bind to PIP2, which localize active zones to PIP2-rich areas of the plasma membrane [9]; and vi) the elucidation of the structure of the C1 domain and the discovery that a tryptophane residue in this domain controls its ability to bind to DAG and phorbol esters [10].
A major accomplishment was the elucidation of the structure of a large Munc13-1 fragment including its C1, C2B and MUN domains (image above), which provided key insights into how the C1 and C2B domain domains mediate diacylglycerol- and Ca2+/PIP2-dependent

presynaptic plasticity [11]. The structure suggested a model whereby Munc13-1 bridges the vesicle and plasma membranes in two different orientations. One orientation is close to perpendicular and can promote SNARE complex formation, but also limits the number of SNARE complexes that are formed and hinders fusion, while the other orientation is much more active, facilitating SNARE complex assembly and increasing the release probability (panel C; the C2C domain, which is proposed to bind to the vesicle, was not part of the elucidated structure and is shown as a cyan ellipse). Binding of the C1 domain to diacylglycerol and of Ca2+ and PIP2 to the C2B domain are expected to strongly favor the active conformation 11. This model has attracted a lot of attention because it explains a large amount of physiological data accumulated on short-term presynaptic plasticity [12].
While our research has provided a wealth of structural information on Munc13s and RIMs, as well as a first glimpse at the molecular mechanisms underlying presynaptic plasticity, there is much to learn about how the functions of the multiple domains of Munc13s and RIMs are integrated within the full-length proteins. Moreover, little is known about the structural organization of the active zone and about the structures of its components other than Munc13s and RIMs, which include RIM-BP, ELKS, liprin, neurexin, bassoon, piccolo, CASK, veli and Ca2+ channels [13]. Hence, this is a widely open area that will gradually constitute the major focus of our research as the mechanisms underlying SNARE complex assembly, Ca2+ sensing and membrane fusion are elucidated in the next few years. Current efforts are directed at determining the structure of full-length Munc13-1 by cryo-EM and incorporating RIM fragments and Rab3A in our reconstitution assays to study the functional interplay between these proteins. We are also starting to express additional components with the goal of reconstituting the active zone.
References
[1] Rizo, J., and Sudhof, T. C. (2012) The Membrane Fusion Enigma: SNAREs, Sec1/Munc18 Proteins, and Their Accomplices-Guilty as Charged?, Annu. Rev. Cell Dev. Biol 28, 279-308.
[2] Betz, A., Thakur, P., Junge, H. J., Ashery, U., Rhee, J. S., Scheuss, V., Rosenmund, C., Rettig, J., and Brose, N. (2001) Functional interaction of the active zone proteins Munc13-1 and RIM1 in synaptic vesicle priming, Neuron 30, 183-196.
[3] Dulubova, I., Lou, X., Lu, J., Huryeva, I., Alam, A., Schneggenburger, R., Sudhof, T. C., and Rizo, J. (2005) A Munc13/RIM/Rab3 tripartite complex: from priming to plasticity?, EMBO J 24, 2839-2850.
[4] Lu, J., Machius, M., Dulubova, I., Dai, H., Sudhof, T. C., Tomchick, D. R., and Rizo, J. (2006) Structural Basis for a Munc13-1 Homodimer to Munc13-1/RIM Heterodimer Switch, PLoS. Biol 4, e192.
[5] Shin, O. H., Lu, J., Rhee, J. S., Tomchick, D. R., Pang, Z. P., Wojcik, S. M., Camacho-Perez, M., Brose, N., Machius, M., Rizo, J., Rosenmund, C., and Sudhof, T. C. (2010) Munc13 C2B domain is an activity-dependent Ca2+ regulator of synaptic exocytosis, Nat. Struct. Mol. Biol 17, 280-288.
[6] Kaeser, P. S., Deng, L., Wang, Y., Dulubova, I., Liu, X., Rizo, J., and Sudhof, T. C. (2011) RIM proteins tether Ca2+ channels to presynaptic active zones via a direct PDZ-domain interaction, Cell 144, 282-295.
[7] Dai, H., Tomchick, D. R., Garcia, J., Sudhof, T. C., Machius, M., and Rizo, J. (2005) Crystal Structure of the RIM2 C(2)A-Domain at 1.4 A Resolution(,), Biochemistry 44, 13533-13542.
[8] Guan, R., Dai, H., Tomchick, D. R., Dulubova, I., Machius, M., Sudhof, T. C., and Rizo, J. (2007) Crystal structure of the RIM1alpha C2B domain at 1.7 A resolution, Biochemistry 46, 8988-8998.
[9] de Jong, A. P. H., Roggero, C. M., Ho, M. R., Wong, M. Y., Brautigam, C. A., Rizo, J., and Kaeser, P. S. (2018) RIM C2B Domains Target Presynaptic Active Zone Functions to PIP2-Containing Membranes, Neuron 98, 335-349 e337.
[10] Shen, N., Guryev, O., and Rizo, J. (2005) Intramolecular occlusion of the diacylglycerol-binding site in the C1 domain of munc13-1, Biochemistry 44, 1089-1096.
[11] Xu, J., Camacho, M., Xu, Y., Esser, V., Liu, X., Trimbuch, T., Pan, Y. Z., Ma, C., Tomchick, D. R., Rosenmund, C., and Rizo, J. (2017) Mechanistic insights into neurotransmitter release and presynaptic plasticity from the crystal structure of Munc13-1 C1C2BMUN, Elife 6.
[12] Neher, E., and Brose, N. (2018) Dynamically Primed Synaptic Vesicle States: Key to Understand Synaptic Short-Term Plasticity, Neuron 100, 1283-1291.
[13] Sudhof, T. C. (2012) The presynaptic active zone, Neuron 75, 11-25.