Rizo lab

Priming of synaptic vesicles to a readily-releasable state enables the fast speed of neurotransmitter release (< 1 ms) upon arrival of an action potential to a presynaptic terminal. The SNAREs syntaxin, SNAP-25 and synaptobrevin (yellow, green and red in the figures) form a tight four-helix bundle [1,2] that is partially assembled during priming. Fast, Ca2+-triggered membrane fusion is believed to be triggered when full SNARE complex assembly brings the vesicle plasma membranes together (see image above) and to involve a close functional interplay between the SNAREs, synaptotagmin (blue) and complexin (pink). However, the mechanism of membrane fusion and the nature of this interplay remain highly unclear.
Our research showed that the two C2 domains that form most of the cytoplasmic region of synaptotagmin (C2A and C2B) bind three and two Ca2+ ions (orange spheres) through loops at the tips of beta-sandwich structures [3-6]. The resulting change in electrostatic potential [7] induces phospholipid binding, and mutations that decrease or increase the apparent Ca2+ affinity of this activity cause parallel changes in the Ca2+ dependence of neurotransmitter release [8,9]. These findings demonstrated that synaptotagmin is the long-sought Ca2+ sensor that triggers release and that Ca2+-dependent phospholipid binding is key for synaptotagmin function.
We also showed that synaptotagmin function is tightly coupled to complexin [10], that complexin binds to the SNARE complex through a central helix that is extended into an accessory helix [11], and that this accessory helix inhibits neurotransmitter release [12], likely because of steric clashes with the vesicle that hinder membrane fusion [13,13] (panel A on the right). A crystal structure solved by the lab of Axel Brunger showed that synaptotagmin binds to the SNARE complex through the C2B domain [15]. This interaction allows simultaneous binding of the C2B domain to PIP2 on the plasma membrane (salmon spheres in panel A), which was supported by a cryo-EM structure [16], and of the SNARE complex to complexin. The resulting state is expected to inhibit membrane fusion because it hinders full zippering of the SNARE complex in the membrane-proximal region (panel A; note that the C-termini of synaptobrevin and syntaxin should be attached to the vesicle and plasma membranes, as indicated by the dashed lines, and hence the C-terminus of the SNARE cannot be assembled).
While it was thought for over 25 years that Ca2+ stimulates synaptotagmin-SNARE binding, we recently found that Ca2+ actually releases synaptotagmin-1 from membrane-anchored SNARE complexes to induce a tight, specific interaction with PIP2 and the membrane (panel B on the right) [17]. All these observations led us to propose a model whereby the primed state of synaptic vesicle includes an inhibited complex similar to that of panel A but with the four-helix bundle partially assembled. In this model, Ca2+ binding to synaptotagmin releases its inhibitory interaction with the SNAREs and allows cooperation of synaptotagmin with the SNAREs in membrane fusion, perhaps because of the ability of synaptotagmin to bridge two membranes and/or to induce membrane curvature [18].
We are currently devoting intense efforts to test this model by characterizing the putative inhibited complex formed by the SNAREs, synaptotagmin and complexin on a membrane, and investigating how the SNAREs and synaptotagmin induce membrane fusion. For this purpose, we are using a combination of NMR analyses on nanodiscs, cryo-EM studies on proteoliposomes, reconstitution assays and molecular dynamics simulations (see snapshot of the system in the image at the top).

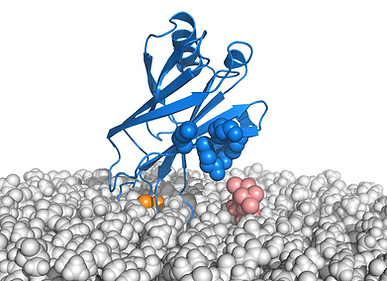
A
B
References
[1] Poirier, M. A., Xiao, W., Macosko, J. C., Chan, C., Shin, Y. K., and Bennett, M. K. (1998) The synaptic SNARE complex is a parallel four-stranded helical bundle, Nat. Struct. Biol 5, 765-769.
[2] Sutton, R. B., Fasshauer, D., Jahn, R., and Brunger, A. T. (1998) Crystal structure of a SNARE complex involved in synaptic exocytosis at 2.4 A resolution, Nature 395, 347-353.
[3] Shao, X., Davletov, B. A., Sutton, R. B., Sudhof, T. C., and Rizo, J. (1996) Bipartite Ca2+-binding motif in C2 domains of synaptotagmin and protein kinase C, Science 273, 248-251.
[4] Ubach, J., Zhang, X., Shao, X., Sudhof, T. C., and Rizo, J. (1998) Ca2+ binding to synaptotagmin: how many Ca2+ ions bind to the tip of a C2-domain?, EMBO J 17, 3921-3930.
[5] Shao, X., Fernandez, I., Sudhof, T. C., and Rizo, J. (1998) Solution structures of the Ca2+-free and Ca2+-bound C2A domain of synaptotagmin I: does Ca2+ induce a conformational change?, Biochemistry 37, 16106-16115.
[6] Fernandez, I., Arac, D., Ubach, J., Gerber, S. H., Shin, O., Gao, Y., Anderson, R. G., Sudhof, T. C., and Rizo, J. (2001) Three-dimensional structure of the synaptotagmin 1 c(2)b-domain. Synaptotagmin 1 as a phospholipid binding machine, Neuron 32, 1057-1069.
[7] Shao, X., Li, C., Fernandez, I., Zhang, X., Sudhof, T. C., and Rizo, J. (1997) Synaptotagmin-syntaxin interaction: the C2 domain as a Ca2+-dependent electrostatic switch, Neuron 18, 133-142.
[8] Fernandez-Chacon, R., Konigstorfer, A., Gerber, S. H., Garcia, J., Matos, M. F., Stevens, C. F., Brose, N., Rizo, J., Rosenmund, C., and Sudhof, T. C. (2001) Synaptotagmin I functions as a calcium regulator of release probability, Nature 410, 41-49.
[9] Rhee, J. S., Li, L. Y., Shin, O. H., Rah, J. C., Rizo, J., Sudhof, T. C., and Rosenmund, C. (2005) Augmenting neurotransmitter release by enhancing the apparent Ca2+ affinity of synaptotagmin 1, Proc. Natl. Acad. Sci. U. S. A 102, 18664-18669.
[10] Tang, J., Maximov, A., Shin, O. H., Dai, H., Rizo, J., and Sudhof, T. C. (2006) A complexin/synaptotagmin 1 switch controls fast synaptic vesicle exocytosis, Cell 126, 1175-1187.
[11] Chen, X., Tomchick, D. R., Kovrigin, E., Arac, D., Machius, M., Sudhof, T. C., and Rizo, J. (2002) Three-dimensional structure of the complexin/SNARE complex, Neuron 33, 397-409.
[12] Xue, M., Reim, K., Chen, X., Chao, H. T., Deng, H., Rizo, J., Brose, N., and Rosenmund, C. (2007) Distinct domains of complexin I differentially regulate neurotransmitter release, Nat. Struct. Mol. Biol 14, 949-958.
[13] Trimbuch, T., Xu, J., Flaherty, D., Tomchick, D. R., Rizo, J., and Rosenmund, C. (2014) Re-examining how complexin inhibits neurotransmitter release, elife 3, e02391.
[14] Prinslow, E. A., Brautigam, C. A., and Rizo, J. (2017) Reconciling isothermal titration calorimetry analyses of interactions between complexin and truncated SNARE complexes, Elife 6, e30286.
[15] Zhou, Q., Lai, Y., Bacaj, T., Zhao, M., Lyubimov, A. Y., Uervirojnangkoorn, M., Zeldin, O. B., Brewster, A. S., Sauter, N. K., Cohen, A. E., Soltis, S. M., onso-Mori, R., Chollet, M., Lemke, H. T., Pfuetzner, R. A., Choi, U. B., Weis, W. I., Diao, J., Sudhof, T. C., and Brunger, A. T. (2015) Architecture of the synaptotagmin-SNARE machinery for neuronal exocytosis, Nature 525, 62-67.
[16] Grushin, K., Wang, J., Coleman, J., Rothman, J. E., Sindelar, C. V., and Krishnakumar, S. S. (2019) Structural basis for the clamping and Ca(2+) activation of SNARE-mediated fusion by synaptotagmin, Nat Commun 10, 2413.
[17] Voleti, R., Jaczynska, K., and Rizo, J. (2020) Ca(2+)-dependent release of Synaptotagmin-1 from the SNARE complex on phosphatidylinositol 4,5-bisphosphate-containing membranes, Elife 9, e57154.
[18] Arac, D., Chen, X., Khant, H. A., Ubach, J., Ludtke, S. J., Kikkawa, M., Johnson, A. E., Chiu, W., Sudhof, T. C., and Rizo, J. (2006) Close membrane-membrane proximity induced by Ca(2+)-dependent multivalent binding of synaptotagmin-1 to phospholipids, Nat. Struct. Mol. Biol 13, 209-217.